FluQS (Flux-based Quantum Speedup)
FLuQS seeks to harness quantum effects required to enhance quantum annealing solutions to hard combinatorial optimization problems. The physics underlying quantum enhancement will be corroborated by design and demonstration of research-scale annealing test beds comprised of novel superconducting qubits, architectures, and operating procedures. All work will serve to demonstrate a plausible path to enhancement and a basis for design of application-scale quantum annealers.
Sponsors: DARPA
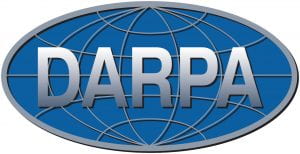
Quantum Limited Sensing
Researchers: Jonathan L. Habif and Arun Jagannathan
Collaborators: Saikat Guha (University of Arizona), Todd Brun (USC), Andrea Armani (USC), Alan Willner (USC), Anupam Madhukar (USC)
Quantum-limited sensing has broad applications in astronomy, communication, and target detection. Optical sensing using traditional techniques such as direct detection or coherent detection is insufficient to extract the maximum amount of information from an optical signal. Hence, the performance of these receivers is severely limited when then are used in a photon starved regime – where the optical signal is very weak. The limitations of an optical receiver that uses conventional detection methods can be overcome by developing new receiver architectures that leverages the power of quantum mechanics. The research mission of this program is to develop new receiver architectures that aims to achieve the fundamental limit of detection – the quantum limit.
Quantum Limited Sensing application: Quantum-limited laser radar detector
An UAV or aircraft can be targeted by a laser radar or laser weapon. By detecting only a few photons of light (photon starved regime), how can the UAV determine whether it is a laser radar/weapon or just background light?
In our recent work, we demonstrated a receiver architecture that approaches the quantum limit of discriminating between a classical state (light from a laser) and thermal state (light from sun or incandescent bulbs) in a photon starved regime (See Figure above). We used our current quantum-limited sensing (QLS) testbed to generate coherent and thermal states at 780 nm and detect them using single photon detectors.
Our current work aims to achieve the following
- Demonstration of quantum optimal receivers to achieve the fundamental limit in measurement precision, the quantum fisher information (QFI) bound for both classical and non-classical states (entangled photons).
- Target detection using non-classical states of light by employing quantum illumination protocol to prevent “spoofing” while detecting targets.
Sponsors: Army Research Office (ARO)
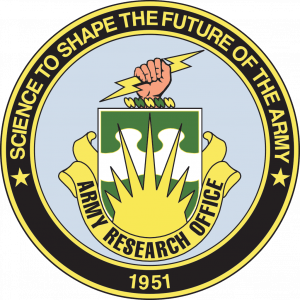
Long Wave Infrared (LWIR) Communication
Researchers: Jonathan L. Habif and Arun Jagannathan
Collaborators: Michelle Povinelli (USC), Steve Cronin (USC)
In this program, we are investigating the use of new materials, with active electrooptic properties in the LWIR wavelength band, to demonstrate a novel free-space optical communications architecture.
The long-wave infrared (LWIR) wavelength band is typically used for passive imaging applications, using cameras with pixels of small bandgap semiconductor heterostructures or bolometers as detectors in a dense focal plane array receiver. This wavelength band also has attractive properties as a free-space communication channel such as high atmospheric transparency and reduced susceptibility to aerosol scattering due to the relatively long wavelength of the light compared with typical optical frequencies used for communications channels. A drawback for using this wavelength band for communications is the omnipresent blackbody background noise that can limit signal to noise ratios at the receiver. In this project, we aim to develop a novel free-space optical communications architecture that uses ubiquitous Planck (‘blackbody’) radiation, inherently generated by all warm objects, as an optical source for a communications signal, and modern LWIR imaging technology as a communications receiver. We recently demonstrated a laboratory-scale communications system using a commercial off-the-shelf LWIR camera comprised of VOx micro-bolometer pixels as the reciever and a quantum cascade laser operating at 10 microns as the transmitter and achieved a transmission rate of 5 bits/s at a distance of 12 meters.
Our current work aims to develop novel materials whose emissivity can be modulated by the application of external voltage.
Sponsors: Department of Defense (DoD)
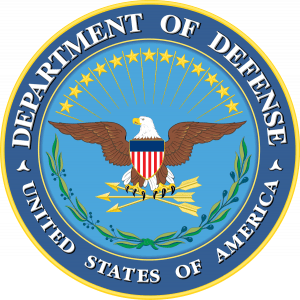
Optical Computing
Researchers: Jonathan L. Habif
Collaborators: Hossein Hashemi (USC), Alan Wilner
In this new project we aim to experimentally demonstrate key components for an all-optical digital signal processor fabricated in a mature, industrial scale integrated photonics fabrication process. This project will validate the core technology to enable self-powered, all-optical digital signal processing micro-systems with the flexibility for a plurality of applications spaces. This investigation is in collaboration with the USC Optical Communications Laboratory (add link) led by Prof. Alan Willner (add link), and the integrated photonics laboratory at USC, led by Prof. Hossein Hashemi.
Sponsors: DARPA
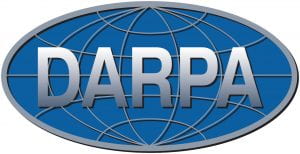
Detection of Atmospherically-Scattered Laser Light
Researchers: Jonathan L. Habif
In this project we are developing novel technical approaches for the passive, off-axis detection of free-space laser light in realistic, daytime ambient background lighting conditions, relevant to passive detection of laser radar or laser weapons. Traditionally, sensitive direct detection receivers have been implemented for the off-axis detection of scattered laser light. The most sensitive direct detection technologies suffer from poor dynamic range, rendering them incapable of detecting weak scattered signals in a bright daylight environment. We are developing and implementing an architecture leveraging coherent detection for the identification of scattered laser light, even in bright daylight conditions. We are building a proof-of-concept demonstration of off-axis detection of scattered c-band (1550nm) laser light, a wavelength commonly used in laser radar systems. Our project is aiming to validate the sensitivity of a passive sensing architecture that can be scaled in optical bandwidth and instantaneous field of view for persistent monitoring for the presence of active laser threats. This project is funded by DARPA.
Sponsors: DARPA
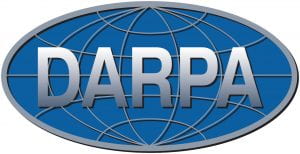
Quantum Simulation
Researchers: Itay Hen, Amir Kalev
Sponsors: Department of Energy (DoE)
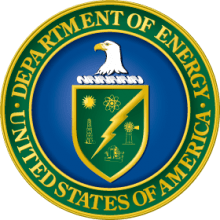
Characterization of Quantum Systems
Researchers: Amir Kalev
Collaborators: Anastasios (Tasos) Kyrillidis, Rice University, and George Kollias, IBM
Sponsors: National Science Foundation (NSF)
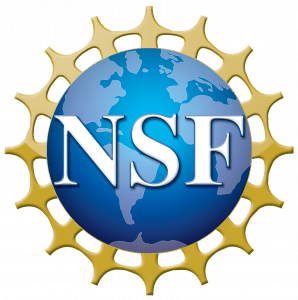